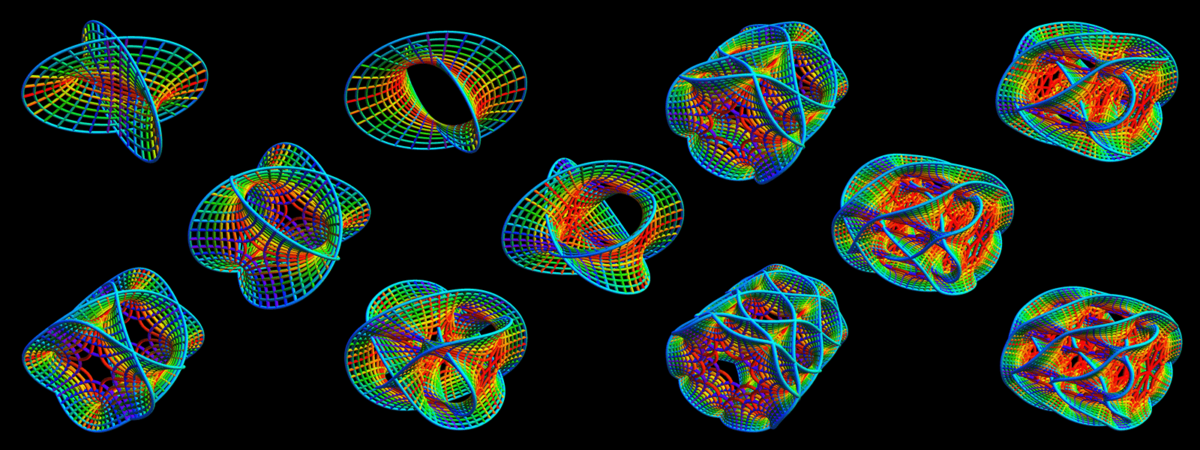
String theory is a mathematical description of nature that requires space to possess several additional dimensions beyond the ordinary three. These extra dimensions, too small to notice in ordinary life, can assume many possible shapes, or geometries (depicted artistically here) that can influence the properties of the universe and subatomic particles. Credit: O. Knill and E. Slavkovsky
Scientists seeking the secrets of the universe would like to make a model that shows how all of nature’s forces and particles fit together. It would be nice to do it with Legos. But perhaps a better bet would be connecting everything with strings.
Not literal strings, of course — but tiny loops or snippets of vibrating energy. And the “fit together” needs to be mathematical, not via properly shaped pieces of plastic. For decades now, many physicists have pursued the hope that equations involving an especially tiny “string” could provide the theory that solves nature’s ultimate subatomic mysteries.
String theory, as it’s called, has acquired a sort of fuzzy cultural acclaim, showing up in popular TV shows like The Big Bang Theory and NCIS. Among physicists, reaction to the theory has been mixed. After several promising bursts of discovery in the 1980s and ’90s, strings fell somewhat out of favor for not delivering on their promises. Among those was providing the proper way to include gravity in the quantum theory of subatomic particles. Another was revealing the math that would show nature’s multiple fundamental forces to be just different offspring of one unified force. Still promises unkept.
Yet during the time since string theory’s retreat from the limelight, a considerable cadre of string devotees have labored to tie all the loose ends together. Success remains elusive, but real progress has been made. Questions plaguing physicists about not only the smallest bits of matter but also the properties of the entire universe may yet yield to string theorists’ efforts.
“Many of the unsolved problems in particle physics and cosmology are deeply intertwined,” write physicists Fernando Marchesano, Gary Shiu and Timo Weigand in the 2024 Annual Review of Nuclear and Particle Science. String theory may provide the path to solving those problems.
Reality’s equations
One major approach in this quest is figuring out whether string theory can explain what is known as the Standard Model of particle physics. Developed in the last part of the 20th century, the Standard Model provides a sort of roster listing all of nature’s basic particles. Some provide the building blocks of matter; others transmit forces between the matter particles, governing how they behave.
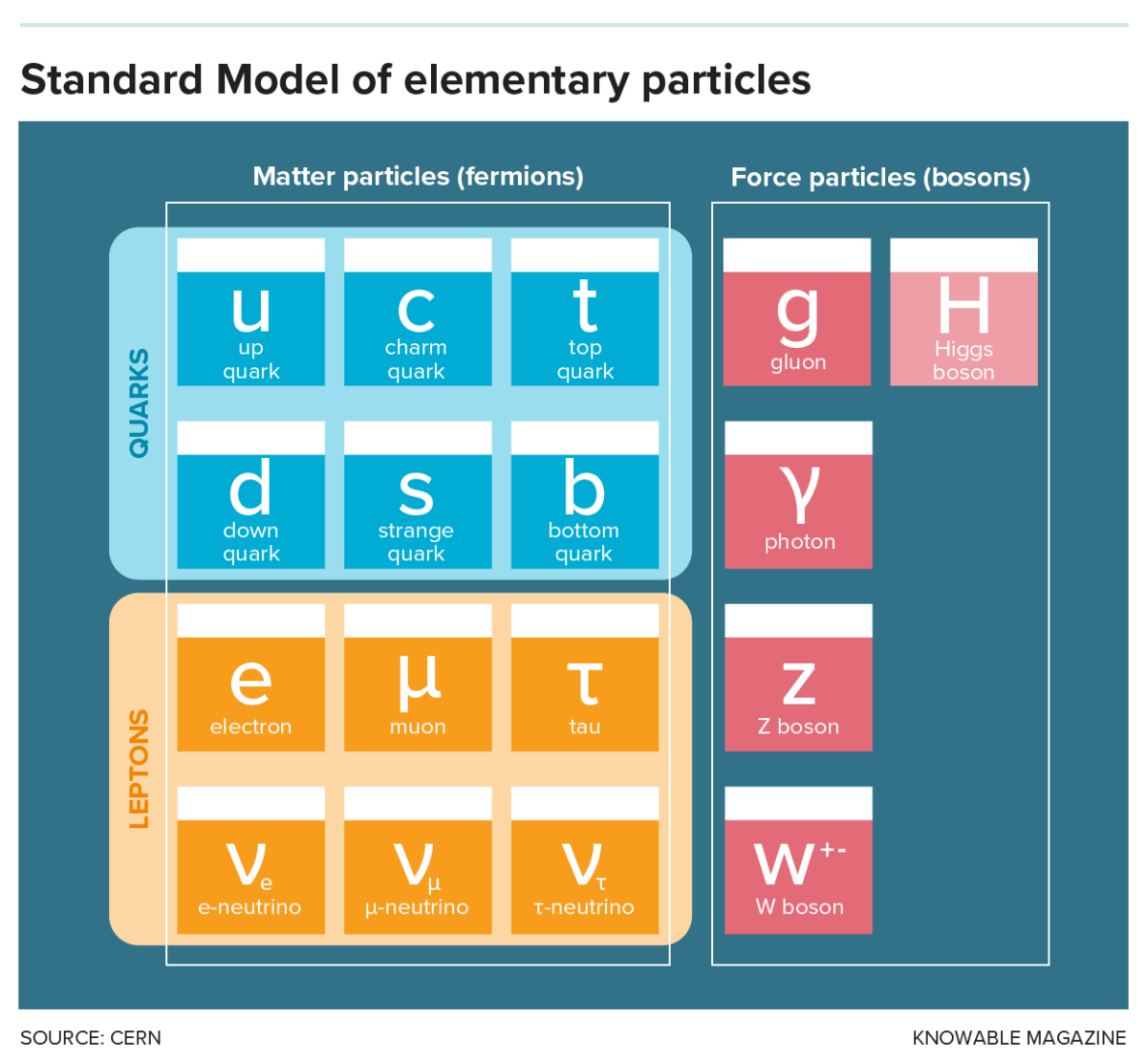
It’s pretty simple to draw a chart displaying those particles. You need 12 spots for matter particles — six quarks and six leptons. You need four spots for force particles (collectively known as bosons) plus a spot for the Higgs boson, a particle needed to explain why some particles have mass. But the mathematics underlying the chart is unfathomably complex, a combination of equations that make hieroglyphics seem self-explanatory.
Those equations work superbly for explaining the results of virtually all particle physics behavior. But the Standard Model cannot be the whole story of the universe. “Despite the incredible success of the Standard Model in describing the observed particle physics up to the currently accessible energy scales, there are compelling arguments for why it is incomplete,” Marchesano and collaborators write.
For one thing, its equations do not encompass gravity, which has no spot on the Standard Model chart. And Standard Model math leaves many questions unanswered, such as why some of the particles have the precise masses that they do. Standard Model math also does not include the mysterious dark matter that lurks within and between galaxies, nor does it explain why empty space is infused with a form of energy that causes the universe to expand at an accelerating rate.
Some physicists investigating these problems believe that string theory can help, since a string version of the Standard Model will contain additional math that could account for its shortcomings. In other words, if string theory is correct, the Standard Model would be just one segment of string theory’s full mathematical description of reality. The problem is that string theory describes many different versions of reality. That’s because the strings exist in a realm with multiple dimensions of space beyond the ordinary three. Kind of like the Twilight Zone on steroids.
String theorists concede that daily life proceeds just fine in a three-dimensional world. Therefore, the extra dimensions of the string world must be too small to notice: They have to shrink, or “compactify,” into submicroscopic size. It’s like the way an ant living on a vast sheet of paper would perceive a two-dimensional surface without ever realizing the paper had a third, very small dimension.
Not only must string theory’s extra dimensions shrink, but they also can shrink into innumerable different configurations, or geometries, of the vacuum of space. One of those possible geometries might be the right shape of the shrunk dimensions to explain the properties of the Standard Model.
“Standard Model … features, questions, and puzzles can be reformulated in terms of the geometry of extra dimensions,” Marchesano and collaborators write.
Because string theory math can be expressed in several different forms, theorists have to explore multiple possible avenues to find the most fruitful formulation. So far, string approaches have been found that describe many features of the Standard Model. But different compactification geometries of the vacuum are needed to explain each feature. The challenge, Marchesano and colleagues point out, is to find one geometry for the vacuum that combines all those features at once, while also incorporating features that describe the known universe.
A successful compactification of the extra dimensions, for instance, would produce a vacuum in space that contained the right amount of “dark energy,” the source of the universe’s accelerating expansion. And candidates for the cosmic dark matter should appear in the string math as well. In fact, a whole additional set of force and matter particles emerges from string equations involving a mathematical property called supersymmetry. “Almost all string theory models that resemble the Standard Model display supersymmetry at the compactification scale,” write Marchesano and his coauthors.
Versions of string theory containing supersymmetric particles go by the moniker “superstring theory.” Such “superparticles” have long been suspected of comprising the universe’s dark matter. But attempts to detect them in space or create them in particle accelerators have so far been unsuccessful.
As for gravity, particles conveying the gravitational force appear naturally in string theory math — one of the theory’s big attractions to begin with. But the fact that many formulations of string theory include gravity does not tell you which formulation provides the correct description of the real world.
Tests are possible
If string theory is correct, fundamental particles of nature would not be the zero-dimensional pointlike objects of standard theory. Instead, different particles would result from different modes of vibration of a one-dimensional string, either a loop or a snippet with ends attached to multidimensional spatial objects called branes. Such strings would roughly be smaller than an atom to the extent that an atom is smaller than the solar system. Very small, with no feasible way of detecting them directly. The amount of energy needed to probe scales so tiny is far beyond the reach of any practical technology.
But if string theory can account for the Standard Model, it would also contain other features of reality that would be accessible to experiments, such as types of particles not included on the Standard Model chart. “String constructions that realize the Standard Model always contain additional sectors … at an energy scale that could be tested in the near future,” Marchesano and colleagues write.
Ultimately, string theory remains a hopeful candidate for putting all the pieces of the cosmic puzzle together. If it works out, scientists could finally unravel the mysteries about how quantum physics’ relation to gravity, and the properties of nature’s particles and forces, are all deeply linked. “String theory,” write Marchesano and colleagues, “has all the ingredients to help us understand this profound connection.”
Tom Siegfried is a science journalist in Avon, Ohio. His book The Number of the Heavens, about the history of the multiverse, was published in 2019 by Harvard University Press.
This article originally appeared in Knowable Magazine, an independent journalistic endeavor from Annual Reviews. Sign up for the newsletter.